The Groundwater Crisis: The Need for New Data to Inform Public Policy
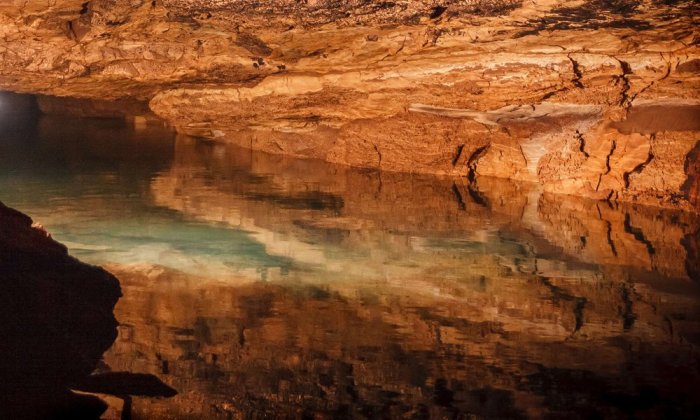
How much groundwater exists and exactly where is it located? How do extreme weather events and climate change impact groundwater resources? In this Effective Policy Learning piece, first published in the journal Harvard Data Science Review, mathematician and engineer Nick Dudley Ward argues that having reliable, accurate, and timely data about valuable groundwater resources will be essential to our ability to respond effectively to climate change.
The presence of groundwater has sustained humanity and agricultural development for millennia. During the 16th century, Leonardo da Vinci presented numerous theories on how the Earth came to be in his “Codex Leicester,” including the most complete record of his reflections on the movement of water. In particular, he was interested in the mechanisms of the movement of water underground, which he supposed moved in a network of veins with surface emanations such as springs, a hugely important source of fresh water at the time. He correctly surmised that there is a great deal of fresh water hidden from sight. Indeed, of the Earth’s fresh water in liquid form, over 95 percent currently is estimated to reside in the ground.
In more recent times, enormous aquifer systems like the Great Artesian Basin in Australia and the aquifers of the Midwest of the United States have supported the inexorable march of agricultural development throughout the 19th and 20th centuries. These enormous aquifers have played the role of global water reinsurer during times of drought. For example, in recent years, California has relied on pumping huge volumes of groundwater to sustain agriculture over multiple years of drought. In a recent study, the authors observe that groundwater supplies two-thirds of California’s water during droughts compared to a third in non-drought conditions. Other countries like the remote Kingdom of Tonga rely existentially on extremely shallow floating groundwater reserves surrounded by ocean and critically exposed to sea-level rise.
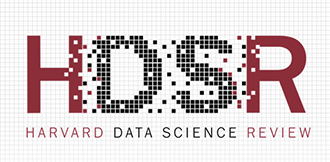
Although many of Earth’s major aquifers are severely depleted, it is hard not to feel that groundwater will become ever more important in the context of climate change. However, the amount of groundwater hidden in Earth’s aquifers remains a huge unknown. We only see the impacts of depletion on a large scale through declining water levels in wells or earth-scale geophysical studies. This lack of data has been harmful in forming clear public policies that can help communities and industries respond effectively to climate change. From a policy perspective, groundwater management is often opaque. As a recent article in The New York Times highlights, there is a lack of consistent regulation between states and within states. In many cases, access to groundwater is simply unregulated or lost in a tangled web of regulations — effectively a free-for-all. This is exacerbated by the lack of basic data, such as not knowing how many wells there are, let alone how much water is being pumped.
In many cases, access to groundwater is simply unregulated or lost in a tangled web of regulations — effectively a free-for-all.
One of the problems associated with detecting changes in groundwater state is that aquifers do not respond in the attention-grabbing way that surface waters do during extreme weather events, when the impacts of floods or droughts are directly observable and immediate, fueling the collective narrative about climate change. Nevertheless, climate models tell us much less about changes in extreme events than they do about changes in the mean state. Even if the current state of groundwater development were to reach a steady state, it is very hard to see how the movement of water in the ground will respond to changes in climatic patterns and, especially, in what manner the greater incidence of extreme weather events will impact the movement of the Earth’s groundwater resources.
Much of the contemporary narrative about data is about having open access to data on appropriately managed databases, and tools to interrogate the data; that is, making data available. In New Zealand, for example, which has a data strategy roadmap, big databases are key agenda topics in government. However, there is less clarity on how certain databases would actually be populated. For example, in the case of groundwater, often data simply does not exist — or is so sparse as not to merit the title of database.
In spite of the lack of data, in recent years shallow groundwater systems have begun to feature in the climate change narrative in New Zealand. These are systems in which the water table ranges from a meter or less below the ground surface to a few meters. In urban environments, they are not usually of interest as a water resource due to pollution but can have substantial impact on the structural health and integrity of buildings as well as underground infrastructure.
The coastal city of Christchurch, New Zealand (pop. 400,000), built on an extensive sequence of artesian aquifers, experienced several major earthquakes between 2010 and 2011 that led to the central business district being substantially rebuilt. During the earthquakes, the city experienced substantial liquefaction in areas where the water table was high: Saturated soil can liquefy and flow (sometimes extensively as in the case of Christchurch) when subjected to strong shaking. In more recent times, with earthquakes replaced by climate change, shallow groundwater systems are being recognized as a different kind of hazard — high water tables together with sea-level rise create many engineering challenges for the built environment from underground infrastructure to resilience of buildings, including a reduction of the potential buffer of the immediate unsaturated subsurface to mitigate the impacts of surface floods.
One positive impact of the Christchurch earthquakes was the creation of a national geotechnical database. Following the earthquakes, thousands of tests were carried out to assess the strength and the response of soils to dynamic and static stresses and over 1,000 piezometers (devices for measuring groundwater levels and pressure) were installed to monitor the water table. Water content is a critical ingredient in determining the strength of a soil. (Groundwater is also a significant part of the underlying fabric of the Earth since it carries stress when confined. There are numerous examples of cities ranging from Boston and Charleston to Mexico City and Jakarta where groundwater pumping has led to significant subsidence.) In areas of Christchurch in which the water table was more than around three meters below the ground surface, there was considerably less damage. For example, water utility damage was 10 times higher in liquefaction areas of Christchurch.
According to John Scott of New Zealand’s Department of Internal Affairs, while “one doesn’t wish a big earthquake on anyone, a silver lining of the Canterbury Earthquakes of 2010–2011 was the creation of the New Zealand Geotechnical Database (NZGD) and a paradigm shift in industry moving towards open data.” Previously, geotechnical information was “hoarded” by engineering consultancies, but stakeholders, including consultancies and clients, bought into the idea of sharing data between the public and private sectors and creating a comprehensive database. The value proposition for engineering consultancies became being a smart user of the database rather than owning a small part of the data.
Extensive monitoring of the water table in Christchurch over the last 10 years has revealed that the shallow groundwater system underlying Christchurch is a highly complex network. It is dynamic and highly responsive to external sources like rainfall events, and other not so obvious drivers, as well as being highly heterogeneous. This is shown by data from 250 automatically monitored piezometers, which also reveal very significant data gaps since the water table can vary significantly over small distances. Other coastal cities in New Zealand have significantly greater uncertainties than Christchurch. Because of the earthquakes, the problem of the water table has been identified and partially monitored in Christchurch. In other New Zealand cities, it is simply surmised.
Characterizing shallow groundwater systems in built environments is a major factor in quantifying insurance risk. As noted above, lower water tables generally lead to more stable foundations. And it is not just earthquake risk — high water tables have many other detrimental consequences on the built environment ranging from damp buildings, groundwater flooding, and subsidence. Benjamin Franklin wrote, “When the well is dry, we know the worth of water.” In our present time he might well be asking for more data on the state of groundwater.
My current work in New Zealand is focused on gathering and analyzing data and creating mathematical models that will characterize shallow groundwater systems in urban environments using seismic and electrostatic techniques, the most common geophysical methods of groundwater exploration. In the former case, excitation signals, or triggers, using explosive charges generate mechanical soundings. In the latter case, electrical excitation signals are passed through the ground to generate electrostatic fields. Signals are recorded on appropriate measurement systems. Statistical inverse problems are then solved to infer the subsurface profile, with volume of water and flow rate being quantities of particular interest. A distinguishing feature of this work is the large number of experiments that will be carried out both at an experimental facility outside Christchurch and within urban areas to create a large database of measurements, with measurement systems, experimental procedures, and computer code to interpret measurement signals developed from the ground up.
One benefit of studying shallow groundwater systems in Christchurch is the network of monitoring piezometers established post-earthquakes that can be used to ground-truth interpreted data. However, as noted above, shallow groundwater systems can be complex, as they are in Christchurch. The complexity is partially offset by modern drilling methods, which allow core samples to be extracted. A key focus of this research is establishing accuracy and repeatability of experiments and interpreted results. This may sound mundane, but it is critical to improve the quality of measurement data and experimental procedures to improve the quality of inference. Accuracy and reliability are critical, because key decisions about urban development (or retreat) may depend on the engineering suitability or challenges of developing a particular area of land.
Getting better at characterizing groundwater systems seems a no-brainer, given our existential predicament.
In sum, this research is about filling large data gaps and reducing significant uncertainties about shallow groundwater systems and their impacts on the ever-increasing built environment, creating the potential for a comprehensive national shallow groundwater database in New Zealand. In parallel, there will be extensive engagement with a broad spectrum of stakeholders ranging from the engineering industry to government departments. Getting better at characterizing groundwater systems seems a no-brainer, given our existential predicament. Shallow groundwater systems are a good place to start.
Groundwater has long been steeped in mystery. Humans have been interested in finding subterranean water for millennia. Water divining is still enthusiastically practiced in many countries — even by federal politicians in Australia. In more modern times scientists have preferred geophysical techniques for subsurface characterization but, especially with traditional methods of interpretation, there are limitations as to the degree of accuracy one can achieve. In the case of groundwater, we would like to be able to comprehensively characterize and monitor the state of a groundwater system. This means being able to identify water-bearing strata and estimate quantities such as the volume of water and the rate of movement to a sufficient degree of accuracy (something some water diviners claim to be able to do). The question remains just how accurately this can be done — or when does a groundwater exploration method become a genuine imaging technology?
It is far from preposterous to suggest that the fate of the human race is inextricably linked to the fate of the Earth’s groundwaters. Da Vinci’s use of the word “veins” to describe subterranean water systems seems apropos. But it is a very complex, multidisciplinary effort to gather, analyze, and understand groundwater data. The Intergovernmental Panel on Climate Change (IPCC), the United Nations body for assessing the science related to climate change, most recently released assessment report AR6, authored by 234 scientists from 64 countries. The report observes that it is hard to differentiate the impacts of intense groundwater development from climate change impacts. In simple terms, we really do not know what is happening to the Earth’s groundwater resources as a result of climate change, since these resources are already under a great deal of stress and effects are masked by the impacts of development. Nevertheless, in the same way that California looks to groundwater during periods of drought, we undoubtedly will look more to the Earth’s groundwaters as global water reinsurer. Having reliable, accurate, and timely data about these valuable groundwater resources will be essential to our ability to respond effectively to climate change.
Nick Dudley Ward is Senior Researcher at Aqualinc Research, Christchurch, Canterbury, New Zealand, and a co-editor of the Effective Policy Learning column at Harvard Data Science Review, where this story was first published.