Artificial Lives: On the Occult Origins of Chemistry and the Stuff of Life
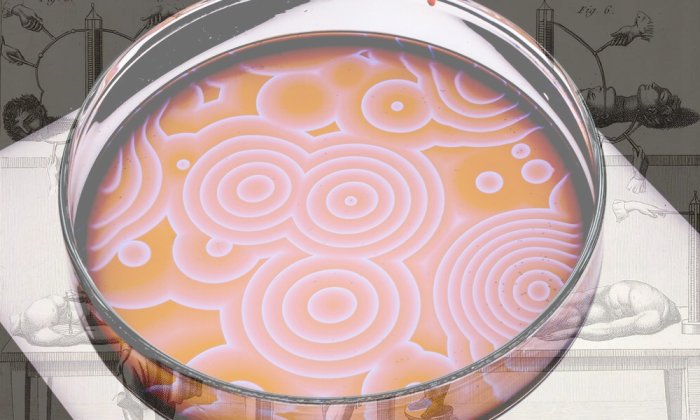
Although it may seem like a modern fantasy, the project of artificially creating a living organism already resounds through the experiments of the ancient alchemists. Long before the birth of modern chemistry, the physician and alchemist Paracelsus, in his 1537 volume “De Rerum Natura,” describes a bizarre biochemical procedure for the preparation of what he called a homunculus, a synthetic organism with the appearance of a very small human being. Paracelsus’s procedure involved the use of certain biological components such as human semen, manure, and blood, which, when subjected to a long process of fermentation, would enable the development of an embryo.
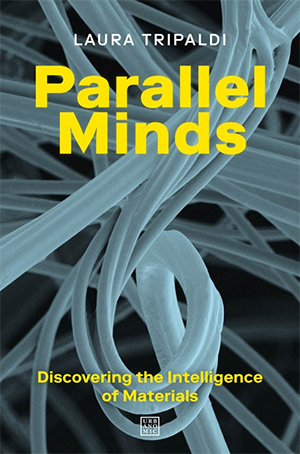
While this type of occult operation may seem absurd today, the result of magical superstitions and, in terms of our contemporary view, a fundamental misunderstanding of how life works, such tales of ancient science often contain deeper and more relevant significance than it may seem. Alchemical lore was based on the idea that the inorganic matter encountered by the alchemist in his laboratory was related to the soul of the human being via a complex of mysterious connections and that it was possible, through scientific investigation, to build a bridge between the chaos of inorganic matter and the harmony of the living body, through experimental work in which chemical transformations were understood to also reflect an inner transformation of the alchemist carrying them out.
Over its long history, chemistry has never entirely freed itself from the ambition to discover how to break through from the inanimate realm of dust and crystals to the domain of the living in all its forms.
I have always been fascinated by the occult origins of chemistry; I think that, although they are not always evident, contemporary chemistry still bears these ancient influences within it. The idea, so dear to alchemists, of a continuity between the inorganic and the organic world, between dead and living matter, was the basis for the development of modern chemistry and still influences many of our technologies today. But in the ancient alchemical legends I believe we also find symbolic confirmation of the idea that, in chemical synthesis, inorganic matter and human beings exert an influence upon one another, forging a fruitful alliance that makes it possible to produce something completely new. Paracelsus’s alchemical procedures, while fascinating, would never have led to any demonstrable result, and yet chemistry, over its long history, has never entirely freed itself from the ambition to discover how to break through from the inanimate realm of dust and crystals to the domain of the living in all its forms.
When Mary Shelley first published “Frankenstein” anonymously in 1818, contemporary science was at a very delicate point. The veil that divided living organisms from inorganic matter was gradually thinning, bringing life and death into dangerously close proximity; increasingly, a certain unexpected continuity was being established between the study of biological organisms and other areas of science and technology. We know that in the writing of her novel Mary Shelley was inspired by recent developments in the science of the times. In 1790 Luigi Galvani had observed by chance that the dissected body of a frog was wracked by intense muscle spasms when the nerves of its legs were touched with an electrostatically charged metal scalpel. Galvani sensed that this response had to have something to do with something inherent to biological organisms, a force he called animal electricity and which, according to the scientist, was transmitted from the brain to the rest of the organism in order to produce movement.
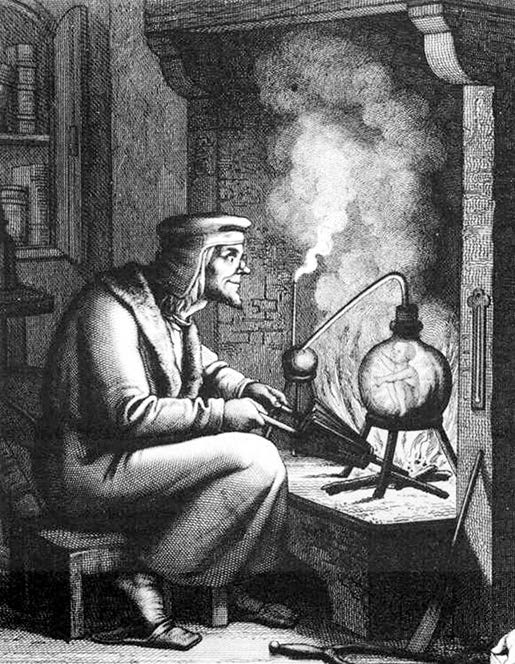
Today we know that the “animal electricity” observed by Galvani is the result of the presence of a difference in electrical potential between the inside and outside of our cells, which is called membrane potential, and is the result of the selective passage of ions through the cell membrane. Galvani could have had no idea of the nature of the phenomenon he was observing — the very notion of electricity, at the time, was still rather unclear, with electrical phenomena being explained by the existence of an “electrical fluid” flowing through conductive bodies — but he understood that the body’s response to the electrical current must have been related to something inside the body itself. Galvani’s experiments also clarified another point: The complex behavior of life was not simply a problem of energy. It was not enough to provide a dead body with energy in just any form, such as heat or mechanical energy, for it to come alive. In order to obtain responses similar to the behavior of a living body, something more specific was needed: a message expressed in physio-chemical language that the tissues of the organism were able to “understand.” This specificity is an inescapable characteristic of living matter: Unlike the rigid and passive bodies imagined by classical physics, which merely transfer energy by bumping into one another, living organisms seem capable of doing something more — transforming external stimuli into a complex response.
Giovanni Aldini, the nephew of Luigi Galvani, took the study of animal electricity, which he called galvanism, to its extremes, applying his uncle’s discovery to the bodies of human beings and exhibiting its miraculous effects in a series of public demonstrations. In his most famous demonstration, which took place in London in 1803, Aldini administered a series of electric shocks to the corpse of a man who had been executed; the man began to come alive, shaken by intense muscular contractions.
Aldini’s aim was to determine the physical origin of that vital force present in all living organisms, and to control it, to the point of crossing the frontier between life and death.
Aldini’s aim was precisely to determine the physical origin of that vital force present in all living organisms, and to control it, to the point of crossing the frontier between life and death. The fame of Aldini’s demonstration undoubtedly contributed to Mary Shelley’s description of Victor Frankenstein’s awakening of the Creature, even though there is no trace of lightning or electric shock in her original story: The role played by electricity in the creation of the monster, so central to the subsequent film adaptations of the work, is actually quite marginal in the novel. The legacy of galvanism in Mary Shelley’s story is instead mainly linked to the fundamental idea that the miracle of life could become accessible to scientific investigation, and that life itself was not alien to the domain of technological action. In short, the truly frightening aspect of crossing the boundary between living and inert matter was not so much the prospect of animating a corpse as the fear of discovering that we ourselves are but animated corpses, governed by the same forces as inorganic matter, yet somehow alive.
Contrary to what might be expected, although the details of the procedure of creation are never entirely revealed to the reader, in Mary Shelley’s imagination Frankenstein’s creation of the monster is more like a process of chemical synthesis than the result of a series of intense electrical shocks. The young Victor Frankenstein, with no access to the most up-to-date theories of natural philosophy, begins his autodidactic “scientific” training following in the footsteps of Renaissance magicians and alchemists such as Paracelsus and Cornelius Agrippa. Upon arriving at university, the young Frankenstein, disappointed by the rigors of a modern science that spurns his dreams of greatness, is driven by a fatal attraction to chemistry, the only scientific discipline that seems capable of fulfilling the miraculous promises of the ancient occult sciences. As his chemistry professor tells him:
The ancient teachers of this science […] promised impossibilities and performed nothing. The modern masters promise very little; they know that metals cannot be transmuted and that the elixir of life is a chimera but these philosophers, whose hands seem only made to dabble in dirt, and their eyes to pore over the microscope or crucible, have indeed performed miracles. They penetrate into the recesses of nature and show how she works in her hiding-places. They ascend into the heavens; they have discovered how the blood circulates, and the nature of the air we breathe. They have acquired new and almost unlimited powers; they can command the thunders of heaven, mimic the earthquake, and even mock the invisible world with its own shadows.
These fatal words, which will lead the young Victor down the road to ruin, seem to suggest that chemistry, despite the apparent humility of its methods, is the only science capable of revealing the secrets of life and death to the devoted scientist. And indeed, throughout its history, chemistry has often found itself wandering the edgelands of life, trying to understand the characteristics that a chemical system must possess in order to be considered living.
As we have seen, the study of complexity reveals that there is no rigid boundary between living and non-living matter: The difference between these two states of matter does not lie in the specific nature of their components, but has to do with different ways in which the same chemical components may be related to one another. What is more, the construction of chemical and nanotechnological systems capable of self-organization shows that, between the almost completely passive behavior of a stone (the chemical structure of which, however, still has a certain degree of complexity) and that of an intelligent animal such as a human, there is a very dense spectrum of different material structures, each capable of interacting in a specific way with the reality around it. Life is first and foremost a problem of organization, and since the dawn of its history, chemistry has always been concerned with understanding how material relates to itself, organizing itself spontaneously into the incredible variety of natural and artificial structures that exist. Even had he lived to the present day, the young Victor Frankenstein would probably have found that the study of chemistry answered best to his ambitions; perhaps, however, his monster would not have been a giant two-and-a-half-metre-tall humanoid, but a microscopic organism a few tens of nanometres across, with a far more alien, if no less threatening, appearance.
Shortly after the publication of “Frankenstein” in 1828, one of the most famous experiments in the history of chemistry was conducted. Studying the synthesis of ammonium cyanate from cyanic acid and ammonia, Friedrich Wöhler observed the unexpected precipitation of a white crystalline substance that had a different appearance from the inorganic salt he had expected to obtain. Wöhler identified this substance as an organic compound known as urea, a molecule naturally present in animal urine; he also noted that the substance he was trying to prepare and the substance he had obtained had the same elementary composition, i.e. they were both made up of the same atoms, but arranged in a different pattern.
This phenomenon, known in chemistry as isomerism, highlights once again the essential importance of structure in determining the behavior of a chemical substance: here too, what separates a “mineral” substance from a “biological” one is not the substance itself, but its organization and structure. Today Wöhler’s experiment is remembered as one of the crucial moments in the development of contemporary chemistry, and is often seen as coinciding with the birth of modern organic chemistry as such: It was one of the first occasions upon which a chemical compound produced spontaneously by living organisms was artificially synthesized in a chemical laboratory from inorganic reactants. Prior to Wöhler’s synthesis, the adjective “organic” was used to connote only substances derived from plants or animals; it is thanks to his experiment that today, in chemistry, the word denotes a specific class of carbon-based chemical compounds, and is now devoid of any necessary association with living organisms.
From Wöhler’s experiment onward, the ability of chemistry to produce substances that are hybrid, i.e. neither entirely inorganic nor entirely living, called into question the rigid separation between life and death but also, and perhaps more significantly, between life and technology. And this unexpected continuity expressed in the synthetic approach of chemistry also emerges in the way in which the development of the chemical sciences has influenced the language we use to talk about matter.
The customary terminology we have used to distinguish the living world from the non-living has always been problematic. Non-living materials have been defined as “inorganic,” “inert,” “inanimate,” or “disorganized,” but each of these terms has ultimately proved inadequate as a way to specify the precise boundaries where life begins: We have synthesized organic materials from inorganic substances and discovered materials that, although not alive, are capable of moving, growing, and remembering. Chemical matter, whether organic or inorganic, is active and dynamic, capable of forming complex organizations on different scales, evolving, and spontaneously modifying its structure in response to the environment. For this reason, rather than considering life as an extraordinary phenomenon, miraculous and extraneous to all other behaviors of matter, chemistry provides us with the tools to think of life as one of the many different types of dynamic organization that matter can assume. In light of these discoveries, it is still possible to provide a definition of life? How can we draw new boundaries for a category that is so elusive, yet so fundamental for us?
We have synthesized organic materials from inorganic substances and discovered materials that, although not alive, are capable of moving, growing, and remembering.
The synthesis of urea is undoubtedly a far more modest result than the one that an ambitious Victor Frankenstein envisioned: The experiment did not lead to the synthesis of an entire organism, it merely demonstrated that certain chemicals present in living organisms can be obtained from inorganic components. However, Wöhler’s result can also be regarded as the first step in a long scientific journey that has brought chemistry closer and closer to an operational understanding of living matter.
In 1862 Louis Pasteur definitively demonstrated the impossibility of the spontaneous generation of living organisms from decaying matter, finally putting paid to a deep-rooted belief that had survived in the history of scientific thought since the time of Aristotle. A new principle was therefore emerging: Life could only be produced from other life, it was not possible for it to emerge from non-living matter. Two opposing tendencies converged: On the one hand, organic chemistry was increasingly effective in demonstrating the ability of inorganic matter to transform, under the appropriate conditions, into the chemical ingredients of life; on the other hand, biology was now convinced that it was impossible to generate life under any circumstances except from an already-formed living being. But then how was it possible for life to have emerged on Earth in the first place?
Pasteur’s dogma, while useful in disproving an unfounded superstition about the spontaneous birth of certain organisms, raised a new barrier between inorganic matter and living matter that was destined to be gradually eroded by new scientific theories of the origin of life. The idea that life originated from non-biological chemical components is known as abiogenesis, and was advocated by the Russian biologist Aleksandr Ivanovič Oparin, who, in his 1924 book “The Origin of Life,” was the first to develop the theory of chemical evolution, i.e. the idea that life emerged from increasingly complex organic molecules. According to Oparin, this approach makes it possible to overcome both the notion that life is a unique and unreproducible phenomenon and the ancient theory of spontaneous generation, rooted in vitalism and equally unviable as an explanation for the origin of life on Earth.
Following in the footsteps of Oparin’s theories, the American chemist Stanley Miller, under the guidance of his professor Harold Urey, developed a crucial experiment in 1953, designed with the aim of demonstrating that abiogenesis was indeed possible, and that the primordial Earth could have furnished appropriate conditions in which life’s constituent molecules could have been spontaneously produced from a set of inorganic ingredients.
Miller developed a simple experimental apparatus consisting of a flask of boiling water in contact with a special gas mixture consisting of ammonia, methane and hydrogen, which, according to the theories of Oparin and Urey, approximated the primordial atmosphere present on Earth at the time when life first emerged. Electrical discharges similar to lightning strikes were then produced within the system by means of two metal electrodes. After a week, Miller purified the aqueous solution he obtained and subjected it to a crude chemical analysis — and confirmed, to his own surprise, that it contained several amino acids, the basic chemical ingredients of living organisms.
Beyond the incredible scientific value of his discovery, there is something romantic in Miller’s experiment — an echo of the 19th-century myth of dead matter animated, in the primordial night of the world, by the power of thunder and lightning.
Laura Tripaldi is a PhD student in Materials Science and Nanotechnology at Università degli Studi di Milano-Bicocca. She is the author of “Parallel Minds,” from which this article is excerpted.