A Complete History of Pandemics
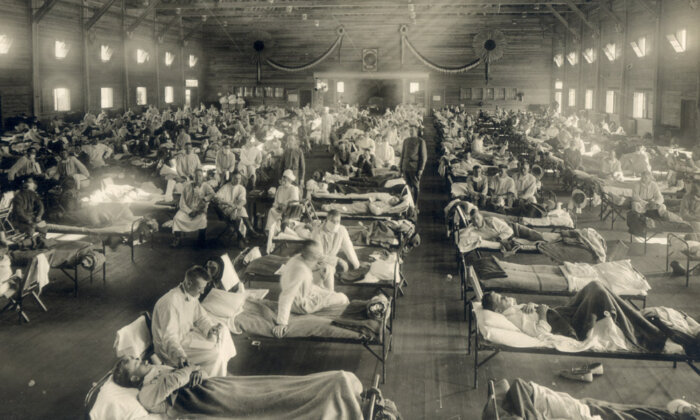
It is still too early to make any reliable judgments as far as the extent of the unfolding Covid-19 pandemic wave is concerned: If official data are correct, it may be dying down in China and it appears to be manageable in South Korea and Taiwan but it is still overwhelming in Italy, Spain, and France and it is in its early exponential phase in the U.S. We cannot be sure if, and with what intensity, the second or even the third wave might come. Over a decade ago, I published “Global Catastrophes and Trends: The Next Fifty Years,” in which I sought not to make forecasts but to provide a close, critical, interdisciplinary look at events that could (in an instant) “change everything” and to offer historical perspectives.
In that book I concluded that another viral pandemic in the near future was certain and that we would be unprepared for it. Of course, I could not have guessed just how unprepared the U.S., so often called the only superpower, would be. In the excerpt featured below, I look at pandemics that ravaged the world.
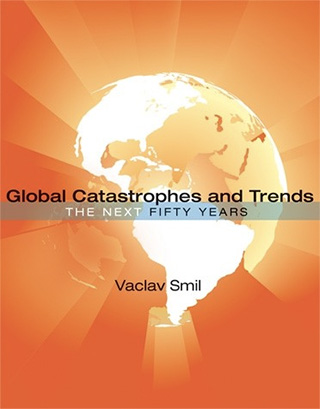
Are we going to learn? We had many opportunities to do so, but as we’re all finding out, we did not take any major steps after the pandemics of 1958-1959, 1968, and 2009. Could it be different this time? Eliminating the risk is impossible but making adequate provisions for the next pandemic is not, and it is a far less costly alternative to scrambling after a crisis arrives. Is it too much to hope that companies would put the security of supply ahead of profits, that people will not insist on ceaseless flying and cruising, and that people accustomed to eating all kinds of wild animals will stop that practice and hence reduce the chances of a new animal virus jumping to people? We will see in a matter of months and years.
Modern hygiene, nationwide and worldwide inoculation, constant monitoring of infectious outbreaks, and emergency vaccinations have either completely eliminated or drastically reduced a number of previously lethal, deeply injurious, or widely discomforting epidemic diseases, including cholera, diphtheria, pertussis, polio, smallpox, tuberculosis, and typhoid. I hasten to add that these have been battles with no assured permanence. Pertussis (whooping cough) is coming back among children too young to be vaccinated. More than 10 million people worldwide still contract tuberculosis every year. The number of multidrug-resistant cases is increasing, and four decades have passed since the introduction of the last new effective anti-TB drugs, rifampicin in 1965 and ethambutol in 1968.
The eradication goal has been particularly elusive in the case of polio. The number of cases dropped worldwide from 350,000 in 1988 to only about 500 in 2001. The next year the number of cases rebounded to some 2,000 a year and after another drop returned to nearly 2,000 in 2005 because of the suspension of vaccination in northern Nigeria, the virus’s persistence in the slums of India, and a sudden increase of infections in Yemen, Somalia, Indonesia.1 In 2005 active transmission of polio virus took place in 16 countries, with endemic presence in Afghanistan, Pakistan, India, and Nigeria, and many polio experts have concluded that, unlike smallpox, the disease cannot be eradicated, only controlled.2
In 1918 it took six days to cross the Atlantic on a liner; now it takes six hours on a jetliner, and there is no doubt that air travel plays an important role in the diffusion of annual epidemics.
A new journal, Emerging Infectious Diseases, published by the Centers for Disease Control and Prevention, is devoted to this global challenge. Since 1975 more than 40 new pathogens (mostly viruses) have been added to the ever-growing list of contagious diseases. They include such scary but limited outbreaks as Ebola hemorrhagic fever in Africa, Nipah virus in Malaysia, Singapore, and Bengal, and hantavirus pulmonary syndrome in the U.S. Southwest as well more widespread and hence more worrisome cases of variant Creutzfeldt-Jakob disease (the human form of mad cow disease, bovine spongiform encephalopathy), cryptosporidiosis, cyclosporiasis, SARS and HIV/AIDS.3
None of these new threats, with the exception of HIV/AIDS, appears capable of changing the course of world history, and AIDS could do so only if new, more virulent strains were to afflict significant shares of populations outside sub-Saharan Africa, where the highest rates of infection now surpass 20 percent and where the disease has its most widespread and most debilitating (social, mental, economic) impacts. During the early 2000s the annual global death toll from AIDS was about 2.8 million people, less than mortality due to diarrhea and tuberculosis, two diseases that we know perfectly well how to eradicate at an acceptably low cost and that now claim annually about 3.4 million lives. Moreover, low and steady rates of HIV infection in many countries, falling rates in some previously badly affected nations (particularly Uganda and Thailand), new multiple drug regimens that extend productive lives (and the hopes for eventual vaccination) show that the disease can be managed.
As far as unpredictable discontinuities are concerned, only one somatic threat trumps all of this: we remain highly vulnerable to another episode of viral pandemic.
As far as unpredictable discontinuities are concerned, only one somatic threat trumps all of this: we remain highly vulnerable to another episode of viral pandemic. High-frequency natural catastrophes have their somatic counterpart in recurrent epidemics of influenza, an acute infection of the respiratory tract caused by serotype A and B viruses belonging to the family Orthomyxoviridae. Influenza epidemics sweep the world annually, mostly during the winter months, but with different intensities. In the United States there are between 250,000-500,000 new cases every year, about 100,000 people are hospitalized, and 20,000 people die (less than 0.01 percent of the U.S. population).
Infection rates are by far the highest in young children (10 to 30 percent annually) and in people over 65 years of age. Influenza pandemics occur when one of the 16 subtypes (H1-H16) of serotype A viruses, different from strains already present in humans, suddenly emerges, rapidly diffuses around the world (usually within six months), and afflicts between 30 percent and 50 percent of people. The illness, with its characteristic symptoms of fever, myalgia, headache, cough, coryza, debilitation, and discomfort, spreads rapidly (latent period is just 1-4 days), and it is often complicated by bacterial or viral pneumonia. The former can be treated by antibiotics, but because there is no treatment for the latter, it becomes a common cause of death during influenza epidemics.
The first fairly well-documented influenza pandemic occurred in 1580, and there have been six known episodes during the last two centuries.4 In 1830-1833 an unknown subtype originated in Russia; in 1836-1837 another unknown subtype originated possibly in Russia; in 1889-1890 subtypes H2 and H3, originated possibly in Russia; in 1918-1919 subtype H1 (despite its common name “Spanish flu”) originated most likely in the United States; in 1957-1958 subtype H2N2 originated in southern China, with total global excess mortality of more than 2 million people; and in 1968-1969 subtype H3N2 originated in Hong Kong, with excess worldwide mortality of about 1 million people. This low death rate was attributable to protection conferred on many people by the 1957 infection. None of the post-1969 epidemics reached virulent pandemic status.5
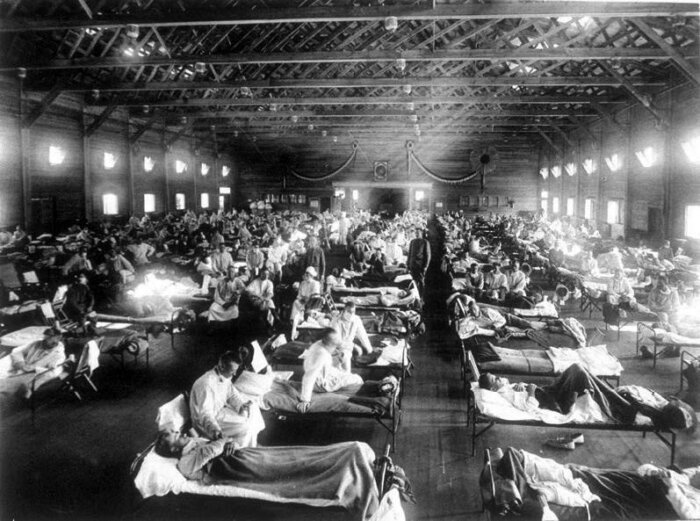
All of the 19th-century pandemics as well as the 1957 and 1968 events were relatively mild and hence did not make any noticeable upticks in the secular trend of declining mortality. By contrast, the 1918-1919 pandemic was by far the largest sudden infectious burden in modern times. A common assumption is that its first, moderately virulent wave began in early March 1918 with the first infections at the U.S. Army Camp Funston in Kansas, but scholar Christopher Langford proposed an origin in China. By May the virus had spread throughout most of the United States, Western Europe, north Africa, Japan, and the eastern coast of China; by August it was in Australia, Latin America, and India. The second wave, between September and December 1918, was responsible for most of the pandemic’s deaths, with mortality as high as 2.5 percent; the third wave (February to April 1919) was less virulent.
Scientific advances of the 1980s (polymerase chain reaction, permitting replication of genetic material) made it possible to identify the virus, which was initially retrieved from formalin-fixed, paraffin-embedded lung tissue samples and used to sequence first the fragments of viral RNA and then the complete genome.6 It characterized the pathogen’s extraordinary virulence. Statistical analyses of the best available data confirm a peculiar mortality pattern: in contrast to annual epidemics characterized by typical U-shaped mortality patterns, the 1918-1919 pandemic inflicted high mortality on people aged 15-35; years; 99 percent of all deaths were in people younger than 65 years. Many of these deaths were due to viral pneumonia, which caused extensive hemorrhaging of the lungs, with death taking place within 48 hours.
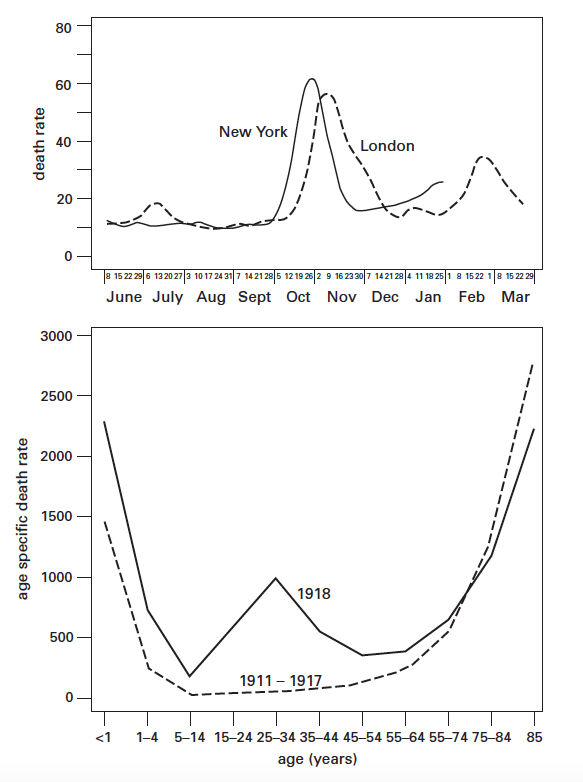
Museum of Health and Medicine, Washington, D. C. Lower, influenza and pneumonia
mortality in the United States, 1911–1917 and 1918.
There is little certainty regarding the total global death toll of the 1918-1919 influenza pandemic. Perhaps the most commonly cited worldwide aggregate 20-40 million, but a key World Health Organization document refers to “upwards of 40 million people,” and the best updated account puts the total at 50 million.7 Even the lowest estimate is higher than all military and civilian casualties of World War I (~15 million). The total of 50 million deaths from the influenza pandemic would be much higher than the global deaths from the great 1347-1351 plague and almost equal the uncertain grand total of deaths among the populations of the world’s two largest Communist regimes of the 20th century, the Stalinist USSR and Maoist China. By any standard, the 1918-1919 influenza pandemic was the deadliest in history. The fairly reliably documented U.S. death toll of 675,000 people was higher than all the deaths sustained by the country’s servicemen in all 20th-century wars.
During the late 1990s, two decades after the last and relatively mild pandemic, new concerns arose because of the emergence of new avian influenza viruses that besides infecting birds and pigs could be transmitted to people. In December 1995 a meeting in Bethesda, Maryland, on pandemic influenza heard from one of the world’s leading experts that “at this time, there is no evidence for or against the direct spread of avian influenza viruses to humans.”8 By the time this presentation was published, the subtype H5N1 mutated in Hong Kong’s poultry markets to a highly pathogenic form, first identified in April 1997, that could kill virtually all affected chickens within two days, and in May 1997 came the first human death, of a three-year-old boy.
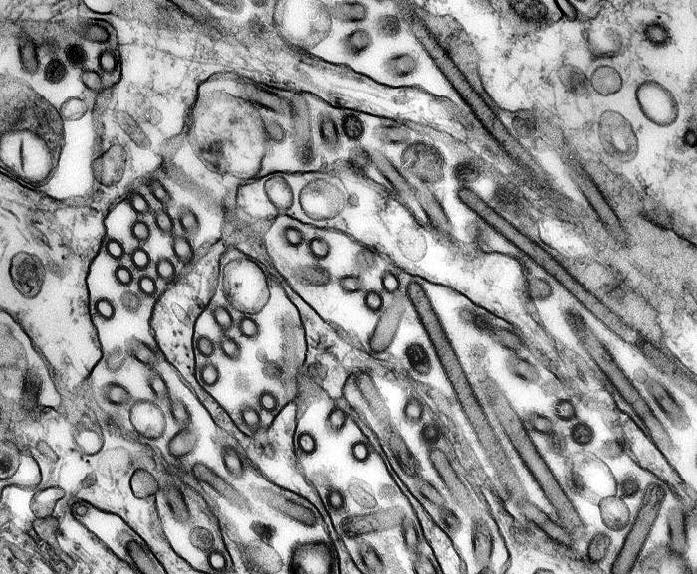
The virus was eventually transferred to at least 18 people, causing six deaths and bringing about the slaughter of 1.6 million birds.9 This episode showed for the first time that avian influenza viruses could infect humans directly, without passing through pigs or other intermediate hosts. Two years later Hong Kong had two poultry-to-people transfers of subtype H9N2, and in 2003 H5N1 strains were isolated from two of the city’s SARS patients. Late in 2003 a highly pathogenic subtype H5N1 began to appear again in poultry in East and Southeast Asian countries. During the next three years the virus was found repeatedly in domestic poultry and wild birds in Japan, South Korea, China, Taiwan, Hong Kong, Vietnam, Laos, Cambodia, Philippines, Indonesia, Malaysia, and Thailand, and it spread westward to Mongolia, Kazakhstan, Turkey, and a number of European countries.
Between December 2003 and the end October 2007 there were 329 laboratory-confirmed cases of human H5N1 infection and 201 deaths, indicating a highly virulent pathogen with mortality of 6 percent. The highest number of infections and deaths were in Indonesia (107, 86), Vietnam (100, 46), and Thailand (25, 17). Fortunately, the H5N1 virus circulating between 2003 and 2006 was not easily transmissible to humans, and the main impact of its spread was economic, necessitating mass slaughter of infected poultry and the stockpiling of vaccines and antiviral medicines. The Thai outbreak in 2004 was particularly widespread, requiring a slaughter of 40 million chickens in 41 provinces.10
There is no way to eliminate the natural reservoirs of this virus. South China’s high densities and ubiquitous proximities of people, poultry, and pigs make the region a perennial source of new viruses, and studies show that domestic ducks in China’s southern provinces are the key reservoir of H5N1. The serotype is now also widely present in wild migratory fowl; ducks, geese, and swans were credited with spreading it across much of Eurasia. However, that assumption may not be correct because there was no such transmission during the eight years after the virus was identified in Hong Kong in 1997, with billions of birds using the same flyways. Poultry shipment and the movement of contaminated materials and wastes may be the primary routes.11
Heightened awareness of the risks posed by H5N1 led epidemiologists to predict a high probability of pandemic influenza in the not-too-distant future.
Because the H5N1 serotype is highly pathogenic and has become ineradicable throughout large parts of Asia, it clearly has a pandemic potential. Heightened awareness of the risks posed by H5N1 led epidemiologists to predict a high probability of pandemic influenza in the not-too-distant future. The following realities indicate the imminence of the risk. The typical frequency of influenza pandemics was once every 50-60 years between 1700 and 1889 (the longest known gap was 52 years, between the pandemics of 1729-1733 and 1781-1782) and only once every 10-40 years since 1889. The recurrence interval, calculated simply as the mean time elapsed between the last six known pandemics, is about 28 years, with the extremes of 6 and 53 years. Adding the mean and the highest interval to 1968 gives a span between 1996 and 2021. We are, probabilistically speaking, very much inside a high-risk zone.
Consequently, the likelihood of another influenza pandemic during the next 50 years is virtually 100 percent, but quantifying probabilities of mild, moderate, or severe events remains largely a matter of speculation because we simply do not know how pathogenic a new virus will be and what age categories it will preferentially attack. Assessing the most likely extent of morbidity and mortality is even more challenging. Despite the enormous advances in virology and epidemiology, many fundamental scientific questions concerning the origins, virulence, and diffusion of influenza remain unanswered. The origin of the 1918-1919 pandemic remains unidentified, and that virulent strain itself was genetically unlike any other known virus examined since that time. Consequently, the current concern about avian H5N1 may be entirely misplaced, whereas a new strain may turn out to be pandemic. We also do not know how influenza A viruses switch to new avian hosts and, most important, what induces them to change so that they can propagate among humans.
Optimistic assessments of the next influenza pandemic put the infection rate at 20 percent of the world’s population, with 1 in every 100 ill people requiring hospitalization (providing the beds will be available) and 7 million deaths in a few months.
The isolated cases of known human-to-human transmissions of H5N1 do not answer that key question. Certainly the most encouraging fact is that the widespread human exposure to infected poultry has produced only a few hundred human infections. This means that the species barrier to H5N1 diffusion is considerable. Moreover, innate immune response (including elevated levels of inflammatory mediators among patients who died) may have been responsible for some of the human disease clusters.
Optimistic assessments of the next influenza pandemic put the infection rate at 20 percent of the world’s population, with 1 in every 100 ill people requiring hospitalization (providing the beds will be available) and 7 million deaths in a few months.12 But the morbidity rate may actually be 25 to 30 percent, and the World Health Organization believes that a new pandemic may affect 20 to 50 percent of the world’s population. Its toll, however, cannot be definitely predicted because we have no way of knowing the eventual virulence of new infectious strains. What is certain is that the appearance of subtype H5N1 has brought us closer to the next pandemic and that, whatever its actual magnitude, we are not adequately prepared for it and for its consequences.
If the eventual death toll were to resemble those of the last two pandemics, with just a few million excess deaths, there would be no global consequences. If it were merely a repeat of the 1918-1919 event, with mortality of no less than 20-25 million and no more than 50 million people, the relative global toll would be obviously much smaller (only about one-fourth as large) than it was four generations ago. But the overall mortality could also be a proportionally potentiated replica of 1918-1919. In the early 2000s we had a 3.4 times larger global population, and an at least eight times larger (nearly 20 billion compared to less than 3 billion) worldwide inventory of poultry (the main reservoir of lethal viruses), with a large share of these birds in large feeding facilities housing tens of thousands of birds.
There are other obvious reasons that could make the next pandemic more costly even if the virulence of the pathogen and the relative mortality rate were no greater than in the 1918-1919 episode. By 2007 the world’s cities, the environment that affords much faster spread of infection, housed 50 percent of all people (76 percent in affluent countries), compared to only about 30% in 1918. Moreover, the global economy is incomparably more integrated, and modern travel and traffic in goods (including live animals and other agricultural products) are several orders of magnitude faster and more voluminous than nine decades ago, a near-ideal condition to spread infections around the world.
The global economy is incomparably more integrated, and modern travel and traffic in goods (including live animals and other agricultural products) are several orders of magnitude faster and more voluminous than nine decades ago, a near-ideal condition to spread infections around the world.
In 1918 it took six days to cross the Atlantic on a liner; now it takes six hours on a jetliner, and there is no doubt that air travel plays an important role in the diffusion of annual epidemics.13 The spread of SARS from Hong Kong to Toronto illustrated how unpredictably and rapidly such diffusions can take place. The extent and intensity of global links also make it impractical to adopt rapid and effective quarantine measures. Additional human factors facilitating the spread of infectious diseases include the rising demand for wild meat (common in both Africa and parts of Asia), drug addiction (including intravenous injections), and mass urban prostitution.
Even assuming “only” 25 million deaths in 1918-1919, we could see a proportionally increased global mortality surpassing 80-100 million people; with 50 million deaths in 1918-1919, the proportional total would rise to 150 to 200 million. With a slightly more than 5 percent mortality rate (that was the well-documented U.S. mean in 1918-1919) there could be at least 1.5 to 2 billion people contracting the infection, and it would be clearly beyond the capacity of health services to cope effectively with such a sudden burden of mass sickness. On the other hand, there are positive factors of generally better nutrition, much better hospital care, and incomparably greater virological understanding. Even so, the overall enormity of ubiquitous morbidity and greatly multiplied mortality would pose challenges unseen in most countries for generations.
In addition, many specific impacts would complicate our ability to deal with immediate challenges and would have long-term consequences. A smaller herald wave of infections several months ahead of the main event (as happened in 1918 in the United States) might not be helpful: rather than giving us more time to prepare, it might actually cause more helplessness and fear because any development of a new vaccine, which would begin only once the pandemic virus started its diffusion, would not be completed before the virus covered the world. But if the pandemic resembled the 1918-1919 episode, then the event might not be over in six months, substantial mortality could continue during the second season, and many cities and countries might find it particularly difficult to cope with the second wave.
If the pandemic resembled the 1918-1919 episode, then the event might not be over in six months, substantial mortality could continue during the second season, and many cities and countries might find it particularly difficult to cope with the second wave.
That phenomenon was illustrated in the case of Toronto’s second wave of SARS in May 2003, minuscule in terms of total numbers but extremely taxing due to mental burdens and logistical problems cause by quarantined hospitals where none but emergency operations were done and no visits, not even to terminally ill patients, were allowed. The mortality burden might shift, as it did so stunningly during the 1918-1919 pandemic, to younger people. Repetition of this pattern could strain the availability and effectiveness of caregivers (health professionals ranging from physicians to staff at retirement homes) and substantially worsen dependency ratios, particularly in Europe’s aging populations, where they have already risen to unprecedented levels.
The massive mortality of people in their prime would also strain the life insurance industry and depress real estate values. And what would the 24-hour news media, so adept at flogging a few accidental deaths in all-day marathons of despair, do with so many deaths that would just keep coming, day after day, week after week? How would the financial markets react to this massive and indiscriminate dying? More important, what would be the long-term economic cost in fear and depression on top of the immediate social and economic insults to the previously insulated Western way of life?
This article is excerpted from “Global Catastrophes and Trends: The Next Fifty Years.”
Vaclav Smil is Distinguished Professor Emeritus at the University of Manitoba. He is the author of over forty books, most recently “Growth.” In 2010 he was named by Foreign Policy as one of the Top 100 Global Thinkers. In 2013 Bill Gates wrote on his website that “there is no author whose books I look forward to more than Vaclav Smil.”
- Roberts, L. 2006. Polio eradication: Is it time to give up? Science 312: 832–835
- Arita, I., M. Nakane, and F. Fenner 2006. Is polio eradication realistic? Science 312: 852–855.
- Morens, D. M., G. K. Folkers, and A. S. Fauci. 2004. The challenge of emerging and reemerging infectious diseases. Nature 430: 242–249.
- Gust, I. D., A. W. Hampson, and D. Lavanchy. 2001. Planning for the next pandemic of influenza. Review in Medical Virology 11: 59–70.
- Kilbourne, E. D. 2006. Influenza pandemics of the 20th century. Emerging Infectious Diseases 12: 9–14.
- Taubenberger, J. K., A. H. Reid, A. E. Krafft, et al. 1997. Initial genetic characterization of the 1918 “Spanish” influenza virus. Science 275: 1793–1796.
- Johnson, N. P., and J. Mueller. 2002. Updating the accounts: Global mortality of the 1918–1920 “Spanish” influenza pandemic. Bulletin of the History of Medicine 76: 105–115.
- Webster, R. G. 1997. Predictions for future human influenza pandemics. Journal of Infectious Diseases 176: S14–S19.
- Snacken, R., et al. 1999. The next influenza pandemic: Lessons from Hong Kong, 1997. Emerging Infectious Diseases 5: 195–203.
- Chotpitayasunondh, T., et al. 2005. Human disease from influenza A (H5N1), Thailand. Emerging Infectious Diseases 11: 201–209.
- Fergus, R., et al. 2006. Migratory birds and avian flu. Science 312: 845.
- Stöhr, K., and M. Esveld. 2004. Will vaccines be available for the next influenza pandemic? Science 306: 2195–2196.
- Grais, R. F., J. H. Ellis, A. Tress, and G. E. Glass. 2004. Modelling the spread of annual influenza epidemics in the U.S.: The potential role of air travel. Health Care Management, Science 7: 127–134.