Could a Self-Sustaining Starship Carry Humanity to Distant Worlds?
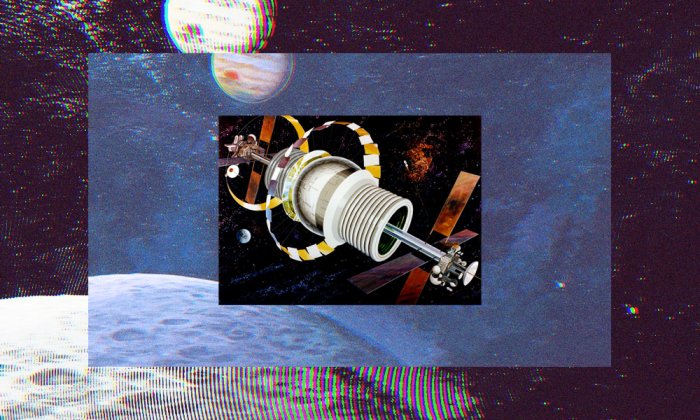
The only barrier to human development is ignorance, and this is not insurmountable.
—Robert Goddard
Until 1992, when the first exoplanets were discovered, there had never been direct evidence of a planet found outside our solar system. Thirty years after this first discovery, thousands of additional exoplanets have been identified. Further, hundreds of these planets are within the “habitable zone,” indicating a place where liquid water, and maybe life, could be present. However, to get there, we need a brave crew to leave our solar system, and an even braver intergenerational crew to be born into a mission that, by definition, they could not choose. They would likely never see our solar system as anything more than a bright dot among countless others.
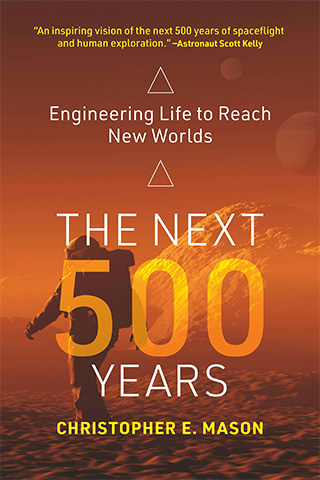
The idea of having multiple generations of humans live and die on the same spacecraft is actually an old one, first described by rocket engineer Robert Goddard in 1918 in his essay “The Last Migration.” As he began to create rockets that could travel into space, he naturally thought of a craft that would keep going, onward, farther, and eventually reach a new star. More recently, the Defense Advanced Research Projects Agency (DARPA) and NASA launched a project called the 100 Year Starship, with the goal of fostering the research and technology needed for interstellar travel by 2100.
This concept of a species being liberated from its home planet was captivating to Goddard, but it has also been the dream of sailors and stargazers since the beginning of recorded history. Every child staring into the night sky envisions flying through it. But, usually, they also want to return to Earth. One day, we may need to construct a human-driven city aboard a spacecraft and embark on a generational voyage to another solar system — never meant to return.
Distance, Energy, Particle Assault
Such a grand mission would need to overcome many enormous challenges, the first and perhaps most obvious being distance. Not including the sun, the closest known star to Earth (Proxima Centauri) is 4.24 light-years, or roughly 25 trillion miles, away. Although 4.24 light-years is a mere hop on the cosmic scale, it would take quite some time to get there with our current technology.
The Parker solar probe, launched by NASA in 2018, is the fastest-moving object ever made by humans, clocking in at 430,000 miles per hour. But even at this speed, it would take 6,617 years to reach Proxima Centauri. Or, put another way, it would take roughly 220 human generations to make the trip.
Using current technology, it would take roughly 220 human generations to make the trip to Proxima Centauri.
The only way to decrease this number would be to move faster. Which brings us to our second challenge: finding the needed energy for propulsion and sustenance. To decrease the amount of time (and the number of generations) it would take to get to the new star, our speed would need to increase through either burning more fuel or developing new spacecraft with technology orders of magnitude better than what is currently at hand. Regardless of the technology used, the acceleration would likely need to come from one or a combination of these sources: prepackaged (nonrenewable) fuel, energy collected from starlight (which would be more challenging when between stars), elements like hydrogen in the interstellar medium, or by slingshotting off of celestial bodies.
The latest advancements in thrust technology might help refocus this issue. Nuclear fusion offers a promising solution, as it produces less radiation and converts energy more efficiently than other methods, which would enable spacecraft to reach much higher speeds. Leveraging nuclear fusion, as envisioned by Project Daedalus (British Interplanetary Society) and Project Longshot (U.S. Naval Academy/NASA), offers a path to interstellar travel within a single human lifetime. These studies suggest that a fusion-powered spacecraft could reach speeds exceeding 62 million miles per hour, potentially reducing travel times to nearby stars to just 45 years.
Yet even if we address the challenges of distance and energy by designing an incredibly fast, fuel-efficient engine, we’re faced with another problem: the ever-present threat of micrometeoroids. Consider that a grain of sand moving at 90 percent of the speed of light contains enough kinetic energy to transform into a small nuclear bomb (two kilotons of TNT). Given the variable particle sizes that are floating around in space and the extremely high velocities proposed for this mission, any encounter would be potentially catastrophic. This, too, would require further engineering to overcome, as the thick shielding we have available to us now would not only degrade over time but would likely be far too heavy. A few solutions might be creating lighter polymers, which can be replaced and fixed as needed in flight; utilizing extensive long-distance monitoring to identify large objects before impact; or developing some kind of protective field from the spacecraft’s front, capable of deflecting or absorbing the impact of incoming particles.
Physiological and Psychological Risks
As exemplified by the NASA Twins Study, the SpaceX Inspiration4 mission, and additional NASA one-year and six-month missions, the crews of a generation ship would face another critical issue: physiological and psychological stress. One way to get around the technological limitation of either increasing the speed of our ships or protecting the ships from colliding with debris is to, instead, slow biology using hibernation or diapause. However, humans who overeat and lie around all day with little movement in simulated hibernation or bed-rest studies can run a higher risk of developing type 2 diabetes, obesity, heart disease, and even death. So, how do bears do it?
During hibernation or torpor, bears are nothing short of extraordinary. Their body temperature dips, their heart rate plummets to as low as five beats per minute, and for months, they essentially do not eat, urinate, or defecate. Remarkably, they’re able to maintain their bone density and muscle mass. Part of their hibernation trick seems to come from turning down their sensitivity to insulin by maintaining stable blood glucose levels. Their heart becomes more efficient as well. A bear essentially activates an energy-saving, “smart heart” mode, relying on only two of its four chambers to circulate thicker blood.
In 2019, a seminal study led by Joanna Kelley at Washington State University revealed striking gene expression changes in bears during hibernation. Researchers used the same Illumina RNA-sequencing technology as used in NASA’s Twins Study to examine the grizzly bears as they entered hyperphagia (when bears eat massive quantities of food to store energy as fat) and then again during hibernation. They found that tissues across the body had coordinated, dynamic gene expression changes occurring during hibernation. Though the bears were fast asleep, their fatty tissue was anything but quiet. This tissue showed extensive signs of metabolic activity, including changes in more than 1,000 genes during hibernation. These “hibernation genes” are prime targets for people who would prefer to wait in stasis on the generation ship than stay awake.
Another biological mechanism that we could utilize on the generation ship is diapause, which enables organisms to delay their own development in order to survive unfavorable environmental conditions (e.g., extreme temperature, drought, or food scarcity). Many moth species, including the Indian meal moth, can start diapause at different developmental stages depending on the environmental signals. If there is no food to eat, as in a barren desert, it makes sense to wait until there is a better time and the rain of nutrients falls.
Diapause is actually not a rare event; embryonic diapause has been observed occurring in more than 100 mammals. Even after fertilization, some mammalian embryos can decide “to wait.” Rather than immediately implanting into the uterus, the blastocyst (early embryo) can stay in a state of dormancy, where little or no development takes place. This is somewhat like a rock climber pausing during an ascent, such as when a storm arrives, then examining all of the potential routes they may take and waiting until the storm passes. In diapause, even though the embryo is unattached to the uterine wall, the embryo can wait out a bad situation, such as a scarcity of food. Thus, the pregnant mother can remain pregnant for a variable gestational period, in order to await improved environmental conditions. The technology to engage human hibernation or diapause doesn’t exist in the 21st century, but one day might.
The impact of weightlessness, radiation, and mission stress on the muscles, joints, bones, immune system, and eyes of astronauts is not to be underestimated. The physiological and psychological risks of such a mission are especially concerning given that the majority of existing models are based on trips that were relatively short and largely protected from radiation by the Earth’s magnetosphere, with the most extensive study so far from Captain Scott Kelly’s 340-day trip.
Artificial gravity — essentially building a spacecraft that spins to replicate the effects of Earth’s gravity — would address many of these issues, though not all. Another major challenge would be radiation. There are a number of ways to try and mitigate this risk, be it shielding around the ship, preemptive medications (actively being studied by NASA), frequent temporal monitoring of cell-free DNA (cfDNA) for the early detection of actionable mutations, or cellular and genetic engineering of astronauts to better protect or respond to radiation. The best defense against radiation, especially in a long-term mission outside of our solar system, would likely be through a combination of these efforts.
But even if the radiation problem is solved, the psychological and cognitive strain of isolation and limited social interaction must be addressed. Just imagine if you had to work and live with your officemates and family, for your entire life, in the same building. While we can carefully select the first generation of astronauts for a long generation ship mission, their children might struggle to adapt to the social and environmental aspects of their new home.
Analog missions performed on Earth have shown that after 500 days in isolation with a small crew, most of the relationships were strained or even antagonistic.
Analog missions performed on Earth, such as the Mars-500 project, have shown that after 500 days in isolation with a small crew, most of the relationships were strained or even antagonistic. There are many descriptions of “space madness” appearing in both fiction and nonfiction, but their modeling and association to risk is limited. There is simply no way to know how the same crew and its descendent generations would perform in 10 or 100 years, and certainly not over thousands of years. Human history is replete with examples of strife, war, factions, and political backstabbing, but also with examples of cooperation, symbiosis, and shared governance in support of large goals (such as in research stations in Antarctica).
Choosing Our New Home
Before we launch the first-ever generation ships, we will need to gain a large amount of information about the candidate planets to which we are sending the first settlers. One way to do this is by sending probes to potential solar systems, gaining as much detail as possible to ensure ships have what they need before they are launched. Work on such ideas has already begun, as with the Breakthrough Starshot mission proposed by Yuri Milner, Stephen Hawking, and Mark Zuckerberg.
The idea is simple enough, and the physics was detailed by Kevin Parkin in 2018. If there were a fleet of extremely light spacecraft that contained miniaturized cameras, navigation gear, communication equipment, navigation tools (thrusters), and a power supply, they could be “beamed” ahead with lasers to accelerate their speed. If each minispacecraft had a “lightsail” targetable by lasers, they could all be sped up to reduce the transit time. Such a “StarChip” could make the journey to the exoplanet Proxima Centauri b — an exoplanet orbiting within the habitable zone of Proxima Centauri — in roughly 25 years and send back data for us to review, following another 25 years of data transit back to Earth. Then, we would have more information on what may be awaiting a crew if that location were chosen. The idea for this plan is credited to physicist Philip Lubin, who imagined in his 2015 article, “A Roadmap to Interstellar Flight,” an array of adjustable lasers that could focus on the StarChip with a combined power of 100 gigawatts to propel the probes to our nearest known star.
The ideal scenario would be seeding the world in preparation for humans, similar to missions being conducted on Mars. If these StarChips work, then they could be used to send microbes to other planets as well as sensors. They certainly have many challenges ahead of them as well, requiring them to survive the trip, decelerate, and then land on the new planet — no small feat. However, this travel plan is all within the range of tolerable conditions for known extremophiles on Earth that casually survive extreme temperatures, radiation, and pressure. The tardigrades, for one, have already survived the vacuum of space and may be able to make the trip to the other planet, and we could have other “seed” organisms sent along, too. Such an idea of a “genesis probe” that could seed other planets with Earth-based microbes, first proposed by Claudius Gros in 2016, would obviously violate all current planetary-protection guidelines, but it might also be the best means to prepare a planet for our arrival. Ideally, this would be done only once robotic probes have conducted an extensive analysis of the planet to decrease the chance of causing harm to any life that may already exist there.
The Ethics of a Generation Ship
These biological, tactical, and psychological issues are driven by one key, last constraint on the generation ship: The passengers are stuck there. As such, this issue represents another challenge that must be addressed: the ethical component. What are the ethics of placing an entire group of people on a single spacecraft, with the expectation that they further procreate additional generations of people, on that ship? They would have to live with the knowledge that the ship on which they live, or are born, is the only world they will ever get to know. Certain social, economic, and cultural infrastructure would need to be built into a generation ship, along with recreational activities.
Bodysuits, virtual/augmented reality camera sets, and immersive experience sets have been built for recreational purposes on Earth, and these would be essential for the generation ship’s crews. Groups could play each other in a virtual environment, which would require less infrastructure than traditional sporting events and equipment do. Video games are, after all, not just exploratory and recreational events; they are a technological glue of society. Of course, games are just a single piece of the puzzle. Life aboard a generation ship would be fundamentally different and undeniably more challenging than anything experienced on Earth.
Some critics of sending spacecraft with humans have argued that if an interstellar mission cannot be completed within the lifetime of the crew, then it should not be started at all. Rather, because the technology for propulsion, design of ships, and rocketry (as well as our methods for genome and biological engineering) will all continue to improve, it would be better to wait. It is even possible that if we sent a generation ship to Proxima Centauri b in the year 2500, it would be passed by another spacecraft with more advanced propulsion sent in the year 3000.
This “incessant obsolescence postulate,” first framed by Robert Forward in 1996, is compelling as a thought experiment. Most technologies do tend to get better, and technology has continued to improve in almost all human societies. So how can one know when the right time is? Predicting the future is notoriously difficult.
The extinction we are trying to avoid could occur in that 500-year lag, resulting in the obliteration of all life with no backup.
However, a good option should not be the enemy of a perfect one. We can send two ships — the first in 2500 and the second in 3000 — not just one. If the new ship catches up to the old one, they would likely be able to assist each other and should plan to do so. Further, this obsolescence concern misses the key risk of waiting too long to act. The extinction we are trying to avoid could occur in that 500-year lag, resulting in the obliteration of all life with no backup.
But even with advanced entertainment and potential hope of a new, enhanced ship appearing any moment, would the crew still stare out the windows into constant star-filled skies thinking of blue oceans? Or would they perhaps be elated about being the “chosen ones” with an extraordinary opportunity to explore and, quite literally, build a new world? The reality is this ship would be their world, and, for most, it would be the only world they would get to experience.
Yet this limitation of experience is actually not that different from the lives of all humans in history. All humans have been stuck on just one world, looking to the stars and thinking, “What if?” This vessel, the Earth, while large and diverse, is still just a single ship with a limited landscape, environment, and resources, wherein everyone up to the 21st century lived and died without the choice to leave. A few hundred astronauts have left Earth, temporarily, but they all had to return. The generation ship is just a smaller version of the one on which we grew up, and, if done properly, it may even be able to lead to a planet that is better than what we inherited. The new planet could be fertile ground for expanding life in the universe, while also offering lessons on how to preserve life on Earth.
Christopher E. Mason is a geneticist and computational biologist who leads the Space Omics and Medical Atlas (SOMA) project and the Cornell Aerospace Medicine Biobank (CAMbank). He is Professor of Genomics, Physiology, and Biophysics at Weill Cornell Medicine, Director of the WorldQuant Initiative for Quantitative Prediction, and the author of “The Next 500 Years: Engineering Life to Reach New Worlds,” from which this article is adapted.