Around the World in 50,000 Years: The Genetics of Race
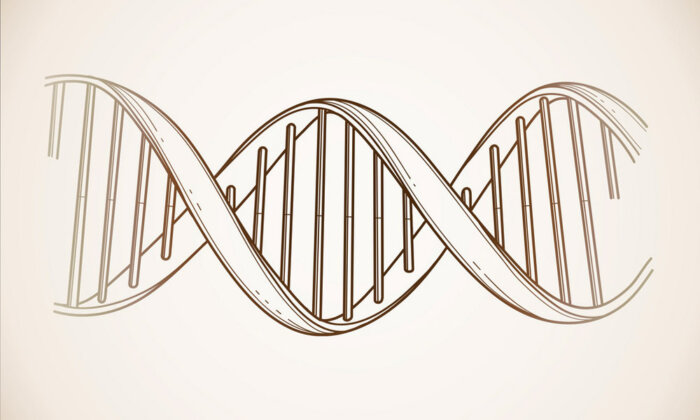
The Food and Drug Administration (FDA) approved BiDil, a drug for the treatment of heart failure in self-identified black patients. . . . “Today’s approval of a drug to treat severe heart failure in [a] self-identified black population is a striking example of how a treatment can benefit some patients even if it does not help all patients,” said Dr. Robert Temple, FDA Associate Director of Medical Policy. “The information presented to the FDA clearly showed that blacks suffering from heart failure will now have an additional safe and effective option for treating their condition. In the future, we hope to discover characteristics that identify people of any race who might be helped by BiDil.”
—FDA News, June 23, 2005
Top-seeded Jimmy Connors stepped onto Centre Court at Wimbledon for the 1975 men’s final having declared that it would be “just another day at the office.” Ranked number one in the world, the 22-year-old defending Wimbledon champion had not dropped a single set en route to the final. The brash left-hander was the overwhelming favorite against the other finalist, sixth-seeded Arthur Ashe. Connors was famed for his explosive outbursts on the court; the 31-year-old Ashe calmly closed his eyes and meditated between games.
The three previous times these rivals had met, Connors had prevailed decisively, and commentators at Wimbledon that day hoped only that Ashe would not be embarrassed on the court. To their surprise, Ashe began the match in dazzling fashion. Instead of trying to out-hit the hard-slugging Connors, Ashe brilliantly executed a game plan of slices, chip returns, lobs, and other change-of-pace shots, to dominate the first two sets 6–1.
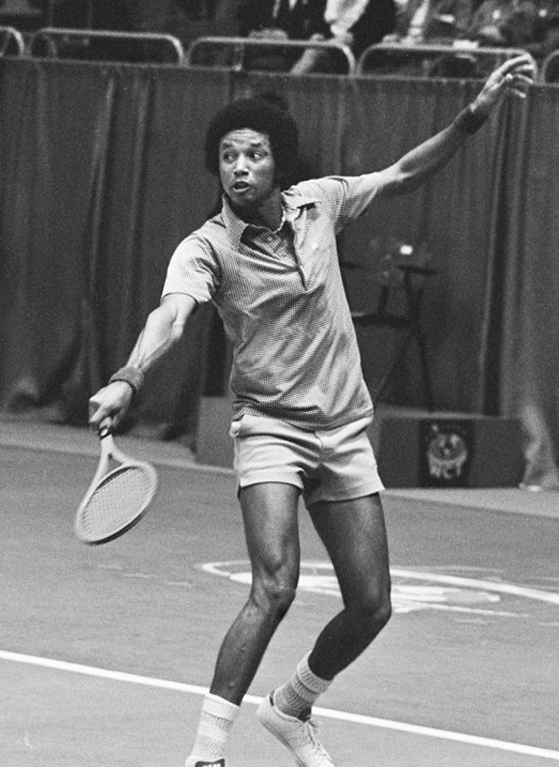
Connors, never a quitter, fought back to win the third set 7–5, keeping alive his hopes for a stirring comeback. He started out the fourth set strongly to gain a 3–0 advantage and was but a point away from 4–1, but Ashe, resolutely sticking to his game plan even when on the defensive, rallied to win the set and match and become the first and still the only African American to win the Men’s Championship of the All England Club. It was the only time he would ever defeat Connors.
Four years after his triumph at Wimbledon, while participating in a tennis clinic, Ashe suffered a heart attack that necessitated quadruple bypass surgery four months later. It forced his retirement from tennis soon after, and his continuing heart problems led to more surgery in 1983.
Ashe never forgot his childhood in segregated Richmond, Virginia, where he had been excluded from whites-only tennis tournaments. Or the Davis Cup match in 1965 between the United States and Mexico that had to be moved from the private Dallas Country Club to a public facility because club members objected to his presence on their courts. He once said that if he was remembered only as a tennis player he would have been a failure. But he is remembered as so much more.
Few issues are as contentious in American society as race. As stellar a citizen of court and country as Ashe was, he was at one time called an Uncle Tom for appearing to legitimize the South African government. At other times he was criticized for not doing enough to further the careers of young black tennis players. Given the history of race in America, the relationship between race and genetics is a landmine for researchers who attempt to study the subject. A host of issues — the very definition of race, the dispute over whether race is a valid categorization of people, the question of which traits might have a race-specific genetic basis, the utility of using racial identity to assist in finding disease genes, and the value of targeting drugs to certain racial groups — provoke intense feelings and heated debate. Because humans seem to have a need to define and differentiate themselves, and because many Americans believe race is so evident a category since it seems to be plainly visible in front of their eyes, the use of race as a classifier of people pervades much of our collective daily existence.
Given the history of race in America, the relationship between race and genetics is a landmine for researchers who attempt to study the subject.
In tackling the issue of genetics and race, we are painfully aware that the widespread racial discrimination in America’s history was often aided by ostensibly objective geneticists claiming to draw on the latest scientific orthodoxy. Efforts from the 17th century onward to classify humans into major groupings perpetuated the notion that the classifiers — invariably white men — belonged to a nobler group than did members of other races. This kind of eugenic thinking culminated in the United States with Jim Crow laws such as the “one-drop” rule, as formulated in the Racial Integrity Act, passed by the Virginia legislature in 1924: “It shall hereafter be unlawful for any white person in this State to marry any save a white person, or a person with no other admixture of blood than white and American Indian. For the purpose of this act, the term ‘white person’ shall apply only to the person who has no trace whatsoever of any blood other than Caucasian” (italics added). This law stood until it was declared unconstitutional by the U.S. Supreme Court in 1967.
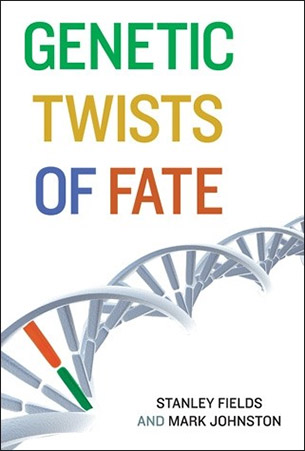
It would be most unfortunate if recent findings from the Human Genome Project and our increasing ability to characterize our personal DNA codes led to a revival of genetic determinism based on racial groupings. Particularly misplaced is the notion that if a genetic association between a disease and a racial group is found, then all members of that group, including individuals who don’t carry the gene variant disposing them to the disease, share the same risk of the disease, especially when the group at risk is not even clearly defined. Even worse is the view that some studies can be construed to support the presumption of a racially determined genetic basis for traits such as athletic ability, intelligence, or criminality, without any good evidence for such a claim.
There is no question that various communities in our society today face enormous disparities in their access to health care, education and employment, and in their diets and levels of stress. Overwhelmingly these disparities boil down not to genetic differences but to economic disadvantages: health is wealth. Yet even when the statisticians account for economic inequality in access to health care and treatments, certain diseases have a much greater prevalence or significantly more severe outcomes in certain populations traditionally viewed as races. Why is this the case?
To understand race and genetics, we have to consider where we came from and how we got here. The fossil evidence suggests that anatomically modern humans, those with physical characteristics not too different from our own, emerged in Africa about 200,000 years ago — an exceedingly brief period in evolutionary time. These humans were part of the lineage of hominids, the family of great apes that comprises humans, chimpanzees, gorillas, and orangutans. The branch of that family that leads to humans split off about six million years ago from the last ancestor we shared with our closest relatives, the chimpanzees; the chimpanzee’s DNA sequence is about 99 percent identical to ours. The human evolutionary tree indicates that humans did not evolve from current chimpanzees (or monkeys or gorillas or apes). Rather, both we and chimpanzees evolved from an ancestor no longer in existence who lived about six million years ago and whose various descendants would eventually give rise to two lineages, one that became us, and one that led to today’s chimpanzees.
Anatomically modern humans first appeared in sub-Saharan Africa, and groups of them ventured out of Africa around 50,000 years ago, spreading throughout the Eurasian landmass — the Mediterranean coast, Europe, Russia, and central Asia — and into Australia. They got to Siberia by 30,000 years ago, and then moved across the Bering Strait and into the Americas about 15,000 years ago, along the way inventing paper in China, mathematics in the Middle East, and country music in the United States. The key point to remember here is that humans spent about 150,000 years in Africa before they colonized the rest of the globe.
Upon their arrival in Eurasia, these early humans likely met the Neanderthals, an abundant hominid species that inhabited Europe and western Asia from about 400,000 to 30,000 years ago. Neanderthals and ancient humans last shared a common ancestor about 500,000 years ago, long before humans walked out of Africa. There is evidence that the globetrotting humans met their Neanderthal cousins in several places, but a comparison of our DNA to theirs suggests that the two groups never got to be very intimate.
Why did it take so long for early humans to venture out of Africa to enjoy the abundance of the rest of the world? It was around the time of the initial migrations out of Africa that humans acquired more sophisticated tools, ornaments, and weapons, even indulging in abstract art, all activities that were evidence of their increased intelligence. This greater brain capacity correlates with a major increase in the population during that time, which may have made them more able to strike out to find new places in the world.
The implications of this model of human evolution are profound. It means that all six-plus billion of us on earth today descended from a small number of people, probably no more than 10,000, who lived in Africa around 50,000 years ago. The migrants who left Africa for points distant were a small subset of all the individuals then alive in Africa, a fact that has far-reaching consequences for human genetics.
If we look at the personal DNA codes of several present-day people to see how many DNA sequence differences we find in them — that is, in how many positions in the genome one person has, say, an A on one strand, and another person has a G — we learn that the number of these variants is significantly greater among Africans than it is among people in other geographic groups. Furthermore, most of the variation seen in populations outside of Africa is also present in the people who live in Africa. For example, if we find that the base at a particular position in the genomes of some Asian people is usually a C and occasionally a T, then we typically find among the African population both the C and the T (and maybe a G as well) at that position. This is because the emigrants brought with them only a sampling of the genetic diversity in the population they left behind. In this case only people with C and T at the position in question emigrated; people with a G at that position stayed behind. Some of the variation reached locations around the globe, but all of it was left behind in the people who stayed back to hold down the fort in Africa. Of course, all humans — those living in Africa as well as those who populated other lands — continued to evolve.
The consequence of a slowly spreading human population is “Race is space,” as Rick Kittles of Howard University and Kenneth Weiss of Pennsylvania State University put it. A new DNA sequence variation that arises in a single individual may spread geographically, but it will move slowly because human generations are long, about 20 years, and in our evolutionary history prior to the advent of internet-based matchmakers we tended to mate only with our neighbors. So if a particular gene variant is found in populations around the globe, it is likely to be ancient, and was probably present 50,000 years ago, when our ancestors hiked out of Africa.
The geographic distance between two populations, the greater their genetic differences.
Conversely, rare variants tend to be much more recent, meaning that they have arisen within the last 50,000 years, and tend to be found only in individuals living in particular regions. In other words, gene variants have been accumulating in people living in Africa for about 200,000 years, much longer than the 50,000 years they have had to accumulate in the population residing on the rest of the planet.
The geographic clustering of early humans did not generate discrete racial groups. Instead, the genetic variation in humans spread in gradients, with the frequency of one particular form of a gene increasing in some directions, decreasing in others. Thus the greater the geographic distance between two populations, the greater their genetic differences: The development of different races is simply due to the space separating them that leads to two genetically distinct populations.
So in light of this history, what is race? There is no generally and consistently accepted definition. Some define a racial group according to physical features such as skin color and hair texture, which reflect a shared ancestry. But others see race as purely an invention, often of white males, to justify cultural practices. Regardless of the conflicting definitions, we think it’s fair to ask whether a biological basis for the concept of race exists.
The key point to bear in mind when discussing possible biological groupings of humans is that no matter what genes you examine and no matter how you define your population groups, about 85 to 95 percent of all the genetic variation you observe in our personal DNA codes is found within all of the population groups; the small balance of variation is all that exists between groups. Imagine that we took random groups of citizens from Cameroon, China, Canada, and the Czech Republic and sequenced all six billion base-pairs of their personal DNA codes (something we’ll be able to do soon). We’d find a lot of differences within each of them — six million or so. But the differences that we’d find among those from Cameroon are very largely the same ones we’d find in common among people belonging to the other three groups. Could we use these sequence data to define a gene or genes for being Cameroonian, or Chinese, or Canadian, or Czech? Of course not! No such genes exist. We are all way too similar in our genetic makeup for that to be possible. Nonetheless, if you compared the variation present in say, the Cameroonian, to the variation present in all the world’s population groups, you would probably find enough specific differences to be able to place that individual quite close to Cameroon.
What about the 5 to 15 percent of the variations that have been found to be typical of one human group or another? Do some of these affect skin color or hair texture or other differences in appearance? Of course they do. All of our physical traits are ultimately determined by our genes. We humans are so anthropocentric that when we look closely at our fellow beings, we notice the tiny differences in the shape of an eye, the slope of a nose, the thickness of a lip. But climb only a hundred feet up a hill and you will have a hard time distinguishing those characteristics. From that perspective we are all nearly identical: the same size, the same shape, with the same number of arms and legs, the same locations for eyes and ears, the same everything else. Thus, classifying individuals into a few groups based on minor differences in appearance and then using those groupings to make inferences about the genetic basis of complex social behaviors is to ignore the huge amount of genetic variation everyone in the world shares.
How do the worldwide patterns of genetic variation that exist affect our ability to identify disease genes? Clearly, some diseases are more prevalent in individuals in one group than in those of another. The prevalence of Tay-Sachs disease is higher in Ashkenazi Jews than in other groups; sickle-cell anemia is most frequent in Africans; phenylketonuria is essentially absent in Africans. “We do not sample Lapps to study Tay-Sachs Disease, Norwegians for sickle cell anemia, or Nigerians for PKU,” write Kittles and Weiss.
So here’s the heart of the race/genetic relationship. Unless and until widespread intermarriage among all humans leads to one homogeneous population, we can more or less divide most of the worldwide pattern of local genetic variation into a few large general, and quite rough, groupings: Africans, Europeans and Middle Easterners, east Asians, and Native Americans. (This oversimplified scheme leaves out a host of smaller subpopulations.) These groupings — which you can call races if you want — contain that small percentage of rare DNA sequence variation (5 to 15 percent) that produces the diversity in the global police lineup.
More important, these rare variants contribute significantly to differences in people’s risk for certain diseases. For example, African American women often develop breast cancer at a younger age than white women who get the disease, and have nearly double the rate of an aggressive form that is resistant to many treatments. Physicians who treat breast cancer patients are beginning to look to Africa to explain some of these differences, hoping to find genetic variants there that may predispose black women to this virulent form of the disease. Another example: Genetic variants among Ashkenazi Jews, a small subgroup of all humans with European origins, lead to an incidence of Tay-Sachs disease 100 times greater than is found in other populations. But these differences in our DNA don’t reflect some kind of inferior genetics, any more than the much higher rate of PKU in people with lighter skin than in people with darker skin says anything about racial fitness.
Using minor differences in appearance to make inferences about the genetic basis of complex social behaviors is to ignore the huge amount of genetic variation everyone in the world shares.
What about BiDil and the targeting of pharmaceuticals to racial groups? This drug is actually a combination of two drugs, hydralazine and isosorbide dinitrate, that had been available for decades and are sold in generic form. Earlier studies of the drug combination had not produced evidence convincing enough to justify its approval, but an analysis of subgroups of patients — a suspect form of data analysis because the question being tested is stated after you have the answer — revealed a benefit of the drug for “blacks.” This finding inspired a new trial called the African-American Heart Failure Trial, carried out only on self-identified African Americans. The results were stunning: BiDil, used along with conventional therapies, led to a 43 percent increase in the rate of survival of heart failure patients compared to those in the study treated only with conventional therapies.
Surely you can appreciate that BiDil does not target the products of genes that influence skin color or hair texture or facial features. Rather, some combination of differences in hypertension, salt sensitivity, and other physiological properties in this self-identified population might differ from the rest of the population such that this drug is especially effective for them. As of this writing, the specific differences in our personal DNA codes that are the basis for this difference aren’t known, but they probably will be soon. At that point, regardless of your skin color or what ethnic group you associate yourself with, if you have the BiDil-sensitive variations in your DNA code, the drug will likely help you. And however dark your skin, or however closely you identify yourself with others with dark skin, if you don’t have those particular DNA sequence variations in your DNA code you won’t be helped by BiDil.
We don’t know whether BiDil would have helped Arthur Ashe after his heart disease became apparent. It likely wouldn’t have mattered anyway. Five years after his second heart surgery, Ashe was hospitalized for toxoplasmosis, a parasitic infection, and learned that he had AIDS, apparently caused by the presence of the Human Immunodeficiency Virus (HIV) in blood he received during his surgery in 1983. Ashe held a press conference in April 1992 to announce that he had the disease. A year later he was dead of AIDS-related pneumonia.
Will the knowledge of the specific DNA sequence variants each of us carry in our personal DNA codes — which affect disease susceptibility, drug efficacy, and many more things that are important to us — end the racism in America that Ashe worked hard to overcome? Will health disparities disappear because we can determine the sequence of DNA and therefore no longer need to classify individuals on the basis of appearance to take advantage of their genetic differences? Likely not. We know all too well that those societal outcomes won’t be realized because of new genetic knowledge. But we can hope that genetic knowledge won’t make the problems any worse.
Someday, perhaps, we’ll come to appreciate that even though the 0.1 percent difference in the DNA between any two of us might mean the difference between being or not being disposed to get a particular disease, the 99.9 percent similarity means that we’re all close relatives: We are all descended from the same ancestors who came out of Africa not so long ago.
Stanley Fields is Professor of Genome Sciences and Medicine at the University of Washington and a Howard Hughes Medical Institute Investigator. Mark Johnston is Professor and Chair of the Department of Biochemistry and Molecular Genetics at the University of Colorado School of Medicine and Editor-in-Chief of the journal Genetics. Fields and Johnston are the authors of “Genetic Twists of Fate.”