In the Animal Kingdom, the Astonishing Power of the Number Instinct
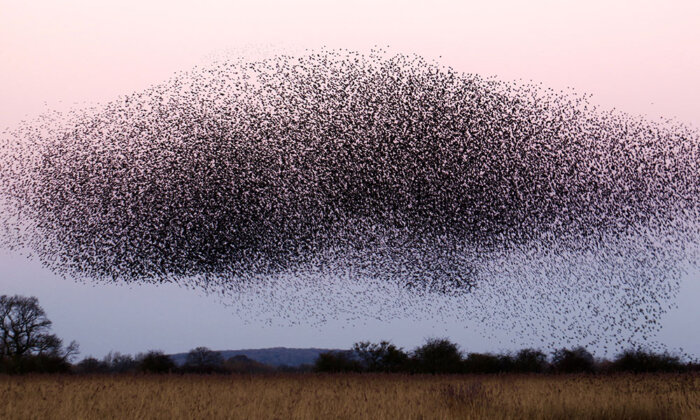
One of the key findings over the past decades is that our number faculty is deeply rooted in our biological ancestry, and not based on our ability to use language. Considering the multitude of situations in which we humans use numerical information, life without numbers is inconceivable. But what was the benefit of numerical competence for our ancestors, before they became Homo sapiens? Why would animals crunch numbers in the first place?
It turns out that processing numbers offers a significant benefit for survival, which is why this behavioral trait is present in many animal populations. Several studies examining animals in their ecological environments suggest that representing number enhances an animal’s ability to exploit food sources, hunt prey, avoid predation, navigate in its habitat, and persist in social interactions.
Staying Alive
Before numerically competent animals evolved on the planet, single-celled microscopic bacteria — the oldest living organisms on earth — already exploited quantitative information. The way bacteria make a living is through their consumption of nutrients from their environment. Mostly, they grow and divide themselves to multiply. However, in recent years, microbiologists have discovered they also have a social life and are able to sense the presence or absence of other bacteria; in other words, they can sense the number of bacteria.
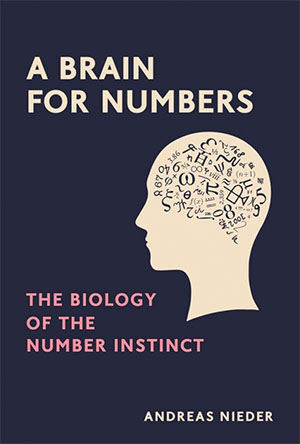
Take, for example, the marine bacterium Vibrio fischeri. It has a special property that allows it to produce light through a process called bioluminescence, similar to how fireflies give off light. If these bacteria are in dilute water solutions (where they are alone), they make no light. But when they grow to a certain cell number of bacteria, all of them produce light simultaneously. Therefore, Vibrio fischeri can distinguish when they are alone and when they are together.
Somehow they have to communicate cell number, and it turns out they do this using a chemical language. They secrete communication molecules, and the concentration of these molecules in the water increases in proportion to the cell number. And when this molecule hits a certain amount, called a quorum, it tells the other bacteria how many neighbors there are, and all bacteria glow. This behavior is called “quorum sensing”: The bacteria vote with signaling molecules, the vote gets counted, and if a certain threshold (the quorum) is reached, every bacterium responds. This behavior is not just an anomaly of Vibrio fischeri; all bacteria use this sort of quorum sensing to communicate their cell number in an indirect way via signaling molecules.
Before numerically competent animals evolved on the planet, single-celled microscopic bacteria — the oldest living organisms on earth — already exploited quantitative information.
Remarkably, quorum sensing is not confined to bacteria; animals are using it to get around, too. Japanese ants (Myrmecina nipponica), for example, decide to move their colony to a new location if they sense a quorum. In this form of consensus decision making, ants start to transport their brood together with the entire colony to a new site only if a defined number of ants are present at the destination site. Only then, they decide, is it safe to move the colony.
Numerical cognition also plays a vital role when it comes to both navigation and developing efficient foraging strategies. In 2008, biologists Marie Dacke and Mandyam Srinivasan performed an elegant and thoroughly controlled experiment in which they found that bees are able to estimate the number of landmarks in a flight tunnel to reach a food source — even when the spatial layout is changed. The honeybee relies on landmarks to measure the distance of a food source to the hive; assessing numbers, then, is vital to its survival.
When it comes to optimal foraging, “going for more” is a good rule of thumb in most cases, and seems obvious when you think about it, but sometimes the opposite strategy is favorable, researchers have found. The field mouse (Apodemus agrarius) loves live ants, but ants are dangerous prey because they bite when threatened. When a field mouse is placed into an arena together with two ant groups of different quantities, then, it surprisingly “goes for less.” In one study, mice that could choose between five versus 15, five versus 30, and 10 versus 30 ants always preferred the smaller quantity of ants. The field mice seem to pick the smaller ant group in order to ensure comfortable hunting and to avoid getting bitten frequently.
Numerical cues play a significant role when it comes to hunting prey in groups, as well. The probability, for example, that wolves capture elk or bison varies with the group size of a hunting party. Wolves often hunt large prey, such as elk and bison, but large prey can kick, gore, and stomp wolves to death. Therefore, there is incentive to “hold back” and let others go in for the kill, particularly in larger hunting parties. As a consequence, wolves have an optimal group size for hunting different prey. For elks, capture success levels off at two to six wolves. However, for bison, the most formidable prey, nine to 13 wolves are the best guarantor of success. Therefore, for wolves, there is “strength in numbers” during hunting, but only up to a certain number that is dependent on the toughness of their prey.
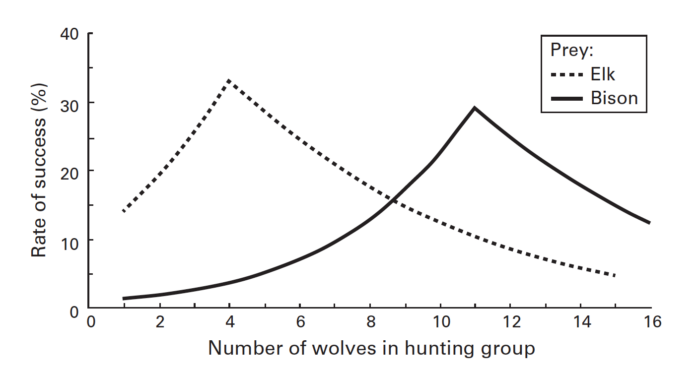
Animals that are more or less defenseless often seek shelter among large groups of social companions — the strength-in-numbers survival strategy hardly needs explaining. But hiding out in large groups is not the only anti-predation strategy involving numerical competence.
In 2005, a team of biologists at the University of Washington found that black-capped chickadees (Poecile atricapilla) in Europe developed a surprising way to announce the presence and dangerousness of a predator. Like many other animals, chickadees produce alarm calls when they detect a potential predator, such as a hawk, to warn their fellow chickadees. For stationary predators, these little songbirds use their namesake “chick-a-dee” alarm call. It has been shown that the number of “dee” notes at the end of this alarm call indicates the danger level of a predator. A call such as “chick-a-dee-dee” with only two “dee” notes may indicate a rather harmless great gray owl. Great gray owls are too big to maneuver and follow the agile chickadees in woodland, so they aren’t a serious threat. In contrast, maneuvering between trees is no problem for the small pygmy owl, which is why it is one of the most dangerous predators for these small birds. When chickadees see a pygmy owl, they increase the number of “dee” notes and call “chick-a-dee-dee-dee-dee.” Here, the number of sounds serves as an active anti-predation strategy.
When chickadees see a pygmy owl, they increase the number of “dee” notes and call “chick-a-dee-dee-dee-dee.” Here, the number of sounds serves as an active anti-predation strategy.
Groups and group size also matter if resources cannot be defended by individuals alone — and the ability to assess the number of individuals in one’s own group relative to the opponent party is of clear adaptive value.
Several mammalian species have been investigated in the wild, and the common finding is that numerical advantage determines the outcome of such fights. In a pioneering study, zoologist Karen McComb and coworkers at the University of Sussex investigated the spontaneous behavior of female lions (Panthera leo) at the Serengeti National Park when facing intruders. The authors exploited the fact that wild animals respond to vocalizations played through a speaker as though real individuals were present. If the playback sounds like a foreign lion that imposes a threat, the lionesses would aggressively approach the speaker as the source of the enemy. In this acoustic playback study, the authors mimicked hostile intrusion by playing the roaring of unfamiliar lionesses to residents.
Two conditions were presented to subjects: either the recordings of single female lions roaring, or of groups of three females roaring together. The researchers were curious to see if the number of attackers and the number of defenders would have an impact on the defender’s strategy. Interestingly, a single defending female was very hesitant to approach the playbacks of a single or three intruders. However, three defenders readily approached the roaring of a single intruder, but not the roaring of three intruders together.
Obviously, the risk of getting hurt when entering a fight with three opponents was foreboding. Only if the number of the residents was five or more did the lionesses approach the roars of three intruders. In other words, lionesses decide to approach intruders aggressively only if they outnumber the latter — another clear example of an animal’s ability to take quantitative information into account.
Our closest cousins in the animal kingdom, the chimpanzees (Pan troglodytes), show a very similar pattern of behavior. Using a similar playback approach, Michael Wilson and coworkers from Harvard University found that the chimpanzees behaved like military strategists. They intuitively follow equations used by military forces to calculate the relative strengths of opponent parties. In particular, chimpanzees follow predictions made in Lanchester’s “square law” model of combat. This model predicts that, in contests with multiple individuals on each side, chimpanzees in this population should be willing to enter a contest only if they outnumber the opposing side by a factor of at least 1.5. And that is precisely what wild chimps do.
Benefits for Reproduction
Staying alive — from a biological stance — is a means to an end, and the aim is the transmission of genes. In mealworm beetles (Tenebrio molitor), many males mate with many females, and competition is intense. Therefore, a male beetle will always go for more females in order to maximize his mating opportunities. After mating, males even guard females for some time to prevent further mating acts from other males. The more rivals a male has encountered before mating, the longer he will guard the female after mating. It is obvious that such a behavior plays an important role in reproduction and therefore has a high adaptive value. Being able to estimate quantity has improved males’ sexual competitiveness. This may in turn be a driving force for more sophisticated cognitive quantity estimation throughout evolution.
One may think that everything is won by successful copulation. But that is far from the truth for some animals, for whom the real prize is fertilizing an egg. Once the individual male mating partners have accomplished their part in the play, the sperm continues to compete for the fertilization of the egg. Since reproduction is of paramount importance in biology, sperm competition causes a variety of adaptations at the behavioral level.
Male pseudoscorpions smell the number of competitor males that have copulated with a female and adjust by progressively decreasing sperm allocation as the number of different male olfactory cues increases from zero to three.
In both insects and vertebrates, the males’ ability to estimate the magnitude of competition determines the size and composition of the ejaculate. In the pseudoscorpion (Cordylochernes scorpioides), for example, it is common that several males copulate with a single female. Obviously, the first male has the best chances of fertilizing this female’s egg, whereas the following males face slimmer and slimmer chances of fathering offspring. However, the production of sperm is costly, so the allocation of sperm is weighed considering the chances of fertilizing an egg. Males smell the number of competitor males that have copulated with a female and adjust by progressively decreasing sperm allocation as the number of different male olfactory cues increases from zero to three.
Some bird species, meanwhile, have invented a whole arsenal of trickery to get rid of the burden of parenthood and let others do the job. Breeding a clutch and raising young are costly endeavors, after all. They become brood parasites by laying their eggs in other birds’ nests and letting the host do all the hard work of incubating eggs and feeding hatchlings. Naturally, the potential hosts are not pleased and do everything to avoid being exploited. And one of the defense strategies the potential host has at its disposal is the usage of numerical cues.
American coots (Fulica americana), for example, sneak eggs into their neighbors’ nests and hope to trick them into raising the chicks. Of course, their neighbors try to avoid being exploited. A study in the coots’ natural habitat suggests that potential coot hosts can count their own eggs, which helps them to reject parasitic eggs. They typically lay an average-sized clutch of their own eggs, and later reject any surplus parasitic egg. Coots therefore seem to assess the number of their own eggs and ignore any others.
An even more sophisticated type of brood parasitism is found in cowbirds (Molothrus ater), a songbird species that lives in North America. In this species, females also deposit their eggs in the nests of a variety of host species, from birds as small as kinglets to those as large as meadowlarks, and they have to be smart in order to guarantee that their future young have a bright future. Cowbird eggs hatch after exactly 12 days of incubation; if incubation is only 11 days, the chicks do not hatch and are lost. It is therefore not an accident that the incubation times for the eggs of the most common hosts range from 11 to 16 days, with an average of 12 days. Host birds usually lay one egg per day; once one day elapses with no egg added by the host to the nest, the host has begun incubation. This means the chicks start to develop in the eggs, and the clock begins ticking. For a cowbird female, it is therefore not only important to find a suitable host, but also to precisely time their egg laying appropriately. If the cowbird lays her egg too early in the host nest, she risks her egg being discovered and destroyed. But if she lays her egg too late, incubation time will have expired before her cowbird chick can hatch.
A study in the American coots’ natural habitat suggests that potential coot hosts can count their own eggs, which helps them to reject parasitic eggs.
Clever experiments by David J. White and Grace Freed-Brown from the University of Pennsylvania suggest that cowbird females carefully monitor the host’s clutch to synchronize their parasitism with a potential host’s incubation. The cowbird females watch out for host nests in which the number of eggs has increased since her first visit. This guarantees that the host is still in the laying process and incubation has not yet started. In addition, the cowbird is looking out for nests that contain exactly one additional egg per number of days that have elapsed since her initial visit.
For instance, if the cowbird female visited a nest on the first day and found one host egg in the nest, she will only deposit her own egg if the host nest contains three eggs on the third day. If the nest contains fewer additional eggs than the number of days that have passed since the last visit, she knows that incubation has already started and it is useless for her to lay her own egg. It is incredibly cognitively demanding, since the female cowbird needs to visit a nest over multiple days, remember the clutch size from one day to the next, evaluate the change in the number of eggs in the nest from a past visit to the present, assess the number of days that have passed, and then compare these values to make a decision to lay her egg or not.
But this is not all. Cowbird mothers also have sinister reinforcement strategies. They keep watch on the nests where they’ve laid their eggs. In an attempt to protect their egg, the cowbirds act like mafia gangsters. If the cowbird finds that her egg has been destroyed or removed from the host’s nest, she retaliates by destroying the host bird’s eggs, pecking holes in them or carrying them out of the nest and dropping them on the ground. The host birds better raise the cowbird nestling, or else they have to pay dearly. For the host parents, it may therefore be worth to go through all the trouble of raising a foster chick from an adaptive point of view.
The cowbird is an astounding example of how far evolution has driven some species to stay in the business of passing on their genes. The existing selection pressures, whether imposed by the inanimate environment or by other animals, force populations of species to maintain or increase adaptive traits caused by specific genes. If assessing number helps in this struggle to survive and reproduce, it surely is appreciated and relied on. This explains why numerical competence is so widespread in the animal kingdom: It evolved either because it was discovered by a previous common ancestor and passed on to all descendants, or because it was invented across different branches of the animal tree of life. Irrespective of its evolutionary origin, one thing is certain: Numerical competence is most certainly an adaptive trait.
Andreas Nieder is Professor of Animal Physiology and Director of the Institute of Neurobiology at the University of Tübingen and the author of “A Brain for Numbers,” from which this article is adapted.